Black Holes
Black holes have a profound impact on their environments. As gas falls towards a super-massive black hole its gravitational potential energy can be converted to heat and light, in some instances producing the most powerful sustained energy sources in the Universe. These Active Galactic Nuclei (AGN) can outshine all of the starlight in an entire galaxy, and sometimes produce relativistic outflows known as jets. The feedback between inflowing gas and outflowing jets and radiation plays an important role in regulating structure formation and evolution in the Universe.
We study the environment immediately surrounding black holes using space-based X-ray observatories (including Chandra, XMM-Newton and NuSTAR), in addition to observations at other wavelengths. X-ray spectroscopy of gas deep in the potential well of the black hole, where the effects of strong gravity are important, allows us to probe the properties of the black hole itself. We numerically model the appearance of these systems in X-rays (see Fig. 1). While current X-ray observatories cannot directly image the accretion disk, X-ray spectra can be used to learn about the spacetime (e.g., is the black hole spinning?) and accretion disk close to the black hole (e.g., its density, iron abundance, emissivity profile and ionization state).
X-rays from accretion flows are highly variable, providing valuable additional information about the system that allows some fundamental degeneracies in spectroscopic studies to be broken. We make significant use of the University of Bristol BlueCrystal supercomputer, including its array of GPUs, to model the variability of X-rays from AGN. In particular, we model the disk response to variable X-ray sources located above the disk (e.g., perhaps in a corona, or the base of an outflowing jet). The simplest of these models would represent a flare from an X-ray point source somewhere above the black hole which produces a time-varying illumination pattern that sweeps across the disk that is distorted as a result of the strong gravitational field and the Doppler shift of the disk material (see Fig. 2). These models can be fit to X-ray data to learn about the changing geometry of the X-ray continuum source.
Working in this area
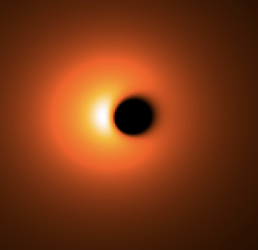
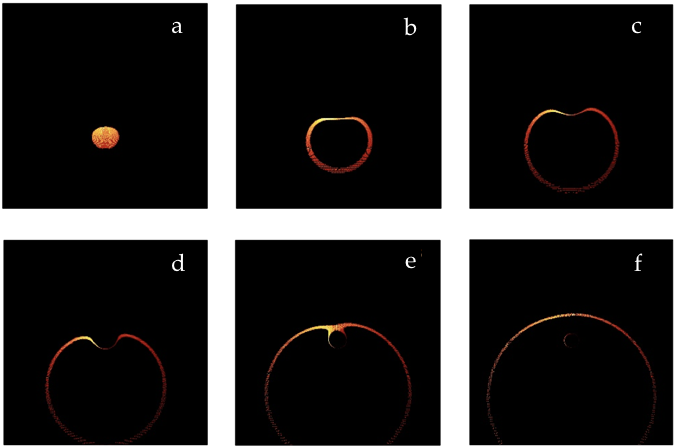